Time—We buy it and spend it, save it and waste it; it seems to race by as we watch our children grow or drag on in the last hour of a Friday work day. Shakespeare marked “time’s thievish progress” in wrinkles like “mouthed graves.” But for a more objective accounting, humans have turned to physics, measuring days, hours, minutes, and seconds using rhythmic natural phenomena such as the Earth’s rotation, the swing of a pendulum, the vibration of quartz crystals, and, most recently, the oscillation of light waves.
“I have been fascinated by time and how we tell it for as long as I can remember,” says Curtis Menyuk, a professor in the Department of Computer Science and Electrical Engineering, who for the past 20 years has applied his expertise in optics to a host of questions at the frontiers of time-keeping science.
Defining time
We measure time in chunks—the international standard unit for a chunk being the second. For centuries, the second was understood as a fraction of an Earth day, but because the Earth’s rotational speed varies slightly, a second defined in this way is not constant. The invention of atomic clocks in the middle of the 20th century allowed scientists to redefine the second based on the (as far as we know) unchanging properties of atoms. Today, one second is the time it takes the electric field of microwaves that are absorbed and emitted by cesium atoms at a specific frequency to cycle up and down 9,192,631,770 times.
But it’s likely the second will soon be redefined again. Newer versions of atomic clocks, called optical clocks, measure time’s passage with visible light instead of microwaves. Visible light waves oscillate much faster than microwaves—and faster oscillations in principle mean better time-keeping. Many optical clocks keep time so well that if they could have started running when the universe began and kept going until today, they still would not have lost or gained a single second.
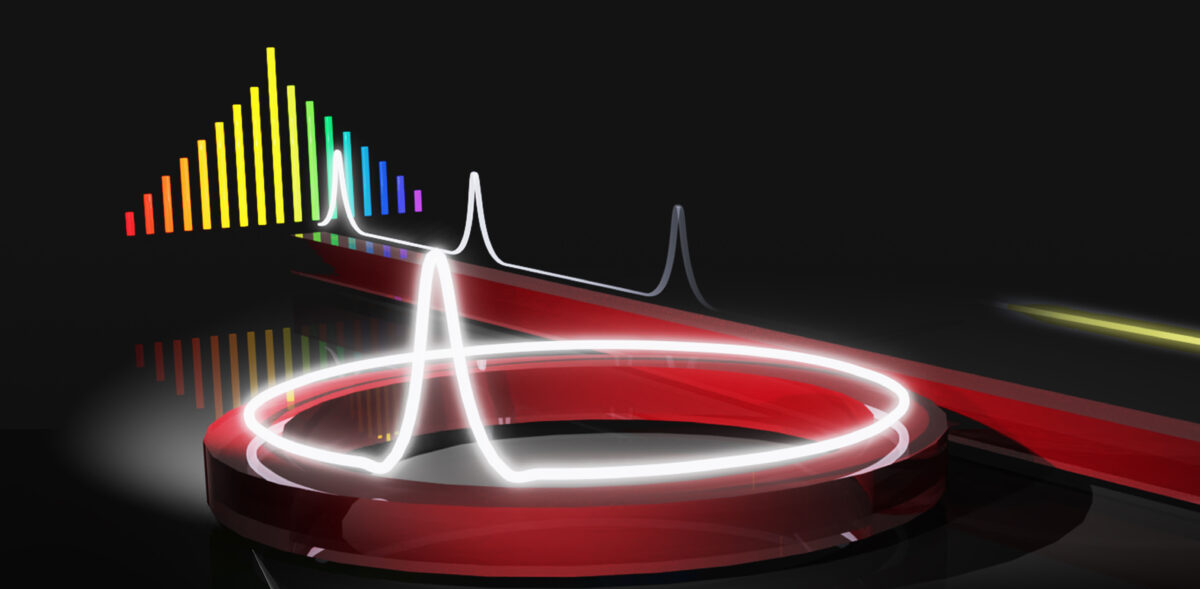
Shortly after the first all-optical atomic clocks were developed in the early 2000s, Menyuk began collaborating with scientists at the National Institute of Standards and Technology (NIST) to study a key component of the clocks called a frequency comb. Frequency combs provide the bridge between high-frequency light, which forms the heart of optical clocks, and modern electronics, which can only process lower-frequency signals. The combs are made from extremely short light pulses, called solitons, that travel without dispersing, similar to how tsunami waves traverse the ocean. And it just so happens that Menyuk is an expert on solitons, having become captivated by the “almost magical balance” of forces that keep the waves from being torn apart ever since he first learned about the phenomenon at the age of 27.
Shrinking the world’s most precise clocks
In 2013, Menyuk collaborated with a colleague to derive the basic equations describing how solitons are created when light travels in a circle around a small crystalline disk called a microresonator. Microresonators offer a compact alternative to the bulky lasers originally required to make frequency combs, and Menyuk and some of his NIST colleagues recently published a paper in the prestigious scientific journal Nature that reports a new approach to simplifying the use and improving the performance of microresonator-based combs.
Shrinking the components of optical clocks is a key step toward taking full advantage of their powers. Their potential extends beyond the possibility of one day setting the time for your computer. Atomic clocks are a critical component of today’s GPS system, which helps billions of people find their way around each day. Compact optical clocks might improve GPS accuracy to within a few centimeters. Optical time-keeping can also serve as a back-up to GPS, which is vulnerable to disruptions and attacks. Menyuk is tackling this very issue with the recent launch of the Center for Navigation, Timing, and Frequency Research at UMBC, which he directs in collaboration with the Army Research Laboratory and other partners.
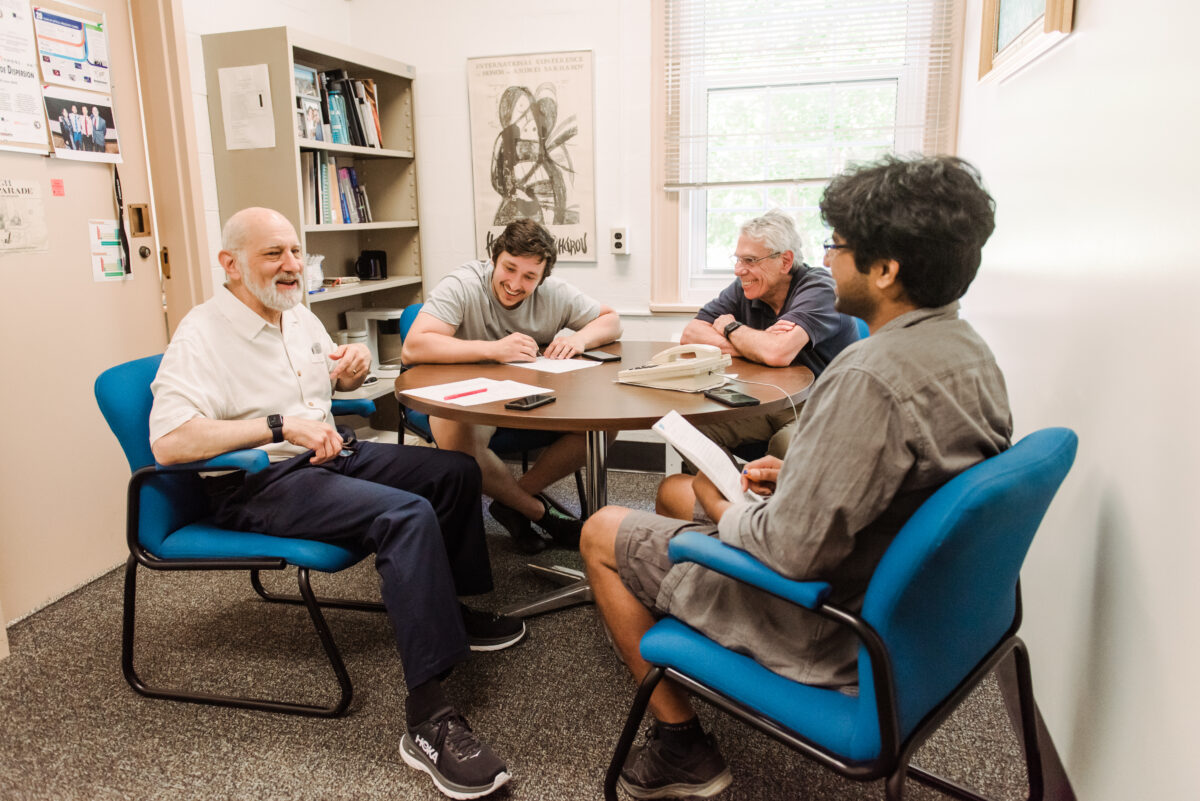
New clocks may even make possible groundbreaking discoveries about the fabric of the universe, helping scientists search for dark matter and test whether the laws of physics change over time.
For Menyuk, passing time has not noticeably dimmed his prolific research output (of which clocks are just a portion). And while the years add age, they also add perspective. “Curtis is a leading theorist with decades of experience,” says Kartik Srinivasan, one of the NIST scientists who collaborated with Menyuk on the recent Nature paper. Srinivasan noted that some of the equations he learned in grad school for predicting light’s behavior in optical fibers were developed by Menyuk in the 1980s. “Curtis’ reservoir of historical knowledge is not something you can easily find.” Even with the use of an atomic clock.
Tags: COEIT, CSEE, Research, Spring 2024