Some organisms are better than others at surviving without oxygen. “Humans don’t do very well without oxygen, but even humans have adaptive mechanisms,” says Rachel Brewster, professor of biological sciences. Zebrafish, however—the model organism Brewster studies—are champs at surviving with little or no oxygen. In fact, zebrafish embryos can last up to 50 hours under anoxia—that is, no oxygen at all.
Brewster’s lab includes wall-to-wall freshwater fish tanks, where two-inch zebrafish with blue and gold horizontal stripes swirl. Her team has been working with zebrafish for years, painstakingly figuring out just how they mitigate the effects of reduced oxygen (“hypoxia”) at a molecular level. “What we’re really interested in discovering is what adaptive molecules we might share in common with some of these highly hypoxia-tolerant organisms like zebrafish,” Brewster says. “And if we share those molecules, how can we control or modify their activity to improve outcomes?”
Solving the zebrafish puzzle
Being able to keep human tissues alive and undamaged under hypoxia for longer stretches of time has a range of potential benefits. Notably, it would expand the ability to deliver donated organs to transplant recipients most in need, in less developed geographic areas, or even in war zones—which is the reason the U.S. Department of Defense (DoD) previously funded Brewster’s work.
Over the last several years, her DoD-funded work and subsequent research supported by the National Institutes of Health (NIH) have led Brewster’s group on a swimmingly successful journey of discovery. Twists and turns, creative thinking, and challenging lab work have allowed them to place one puzzle piece after another to reveal the bigger picture of how zebrafish survive so long without oxygen. Brewster hopes other researchers will take her group’s work a step further, translating the fundamental knowledge they’ve brought forth into treatments that save human lives. In recognition of her group’s contributions, Brewster has just secured a five-year, more than $1.9 million grant from the NIH to continue solving the puzzle.
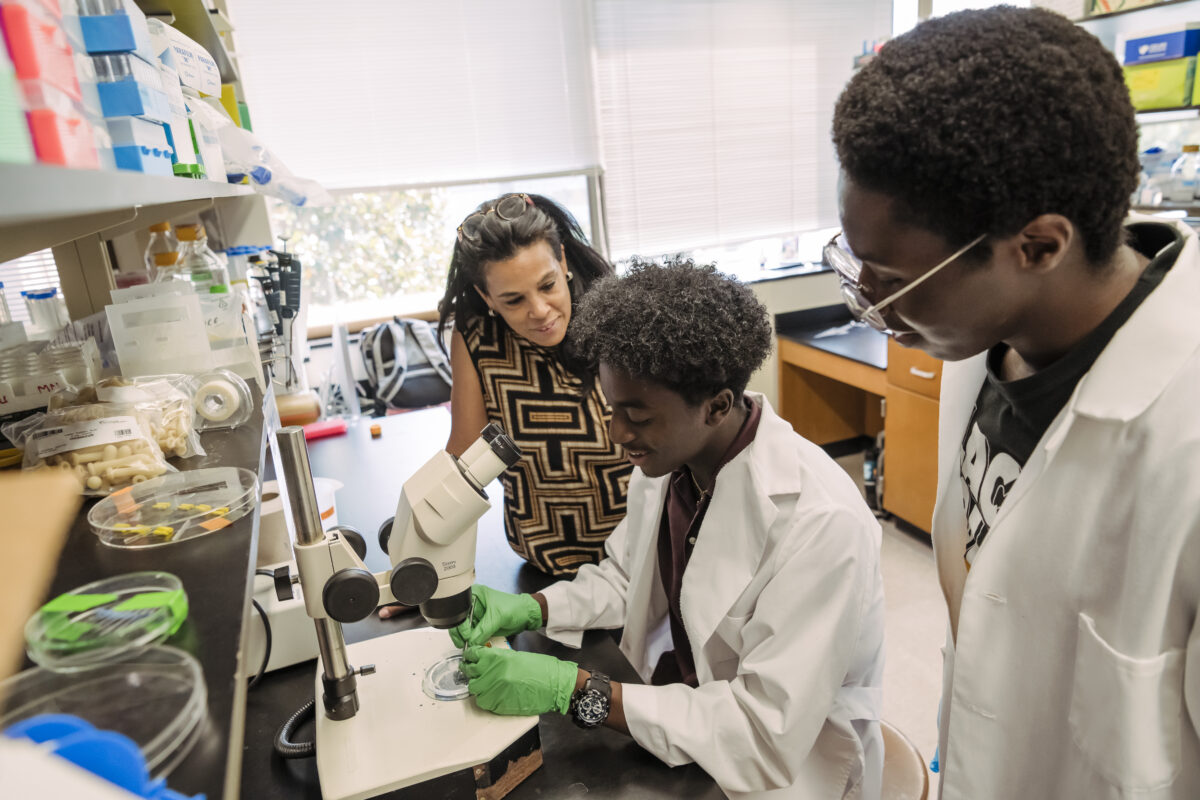
Equally important to Brewster, her research program creates opportunities for emerging scientists to learn the practical skills and habits of mind that will enable them to pursue their own scientific questions in the future. Her current team includes graduate students and several undergraduates, all of whom have contributed to creating new knowledge. “Research and training go together for me,” Brewster says.
The journey begins
Some organisms, including animals that fly at high altitudes, dive deep in the ocean, or live underground, excel at adapting to low oxygen. They typically reduce their metabolic activity when oxygen drops, therefore reducing demand as supply dwindles. “They reach a new status quo,” Brewster says.
Brewster initially expected that the molecules inducing that new status quo must be quick-acting metabolites—small molecules already present in cells—rather than large proteins that are energy-intensive to produce. So in 2016 she teamed up with Johns Hopkins scientist Young-Sam Lee, who has expertise in metabolites. She asked Lee, who is now at the Kentucky University School of Medicine, to identify which metabolites were more or less abundant in zebrafish embryos raised without oxygen compared to embryos that developed under normal conditions. Austin Gabel ’17, biological sciences, worked closely with Lee in summer 2016 to uncover the relevant metabolites.
The results showed that embryos raised without oxygen had, among other metabolites, more lactate. Lactate is a byproduct of glycolysis—the energy-producing process cells must rely on when oxygen isn’t available. That was “kind of a ‘duh’ moment,” Brewster says, but there was more to the story: Around the same time, other researchers were showing that high lactate levels in cancer cells triggered cell growth and blood vessel development via a protein called NDRG3. Brewster was surprised to see such energy-intensive processes triggered by low oxygen—but she wondered if members of the NDRG protein family functioned differently in healthy cells.
A surprising connection
Curious, Brewster and Jong Park, Ph.D. ’21, biological sciences, searched a database for NDRG genes in zebrafish, and found six. (There are four in mammals.) NDRG1a caught their attention, because it is involved in a process that requires an enzyme called a sodium potassium ATPase. That enzyme is one of the most energy-intensive enzymes in the cell, and organisms frequently reduce its production when oxygen is low. Interesting, Brewster thought.
Also, the ATPase and NDRG1a were expressed in exactly the same areas of the fish’s body—the kidney and skin cells called ionocytes, which are arrayed in a polka dot pattern on the surface of zebrafish embryos. Could NDRG1a be regulating the ATPase? Sure enough, a database of protein-protein interactions revealed that NDRG1a could interact with the ATPase. Now they were getting somewhere!
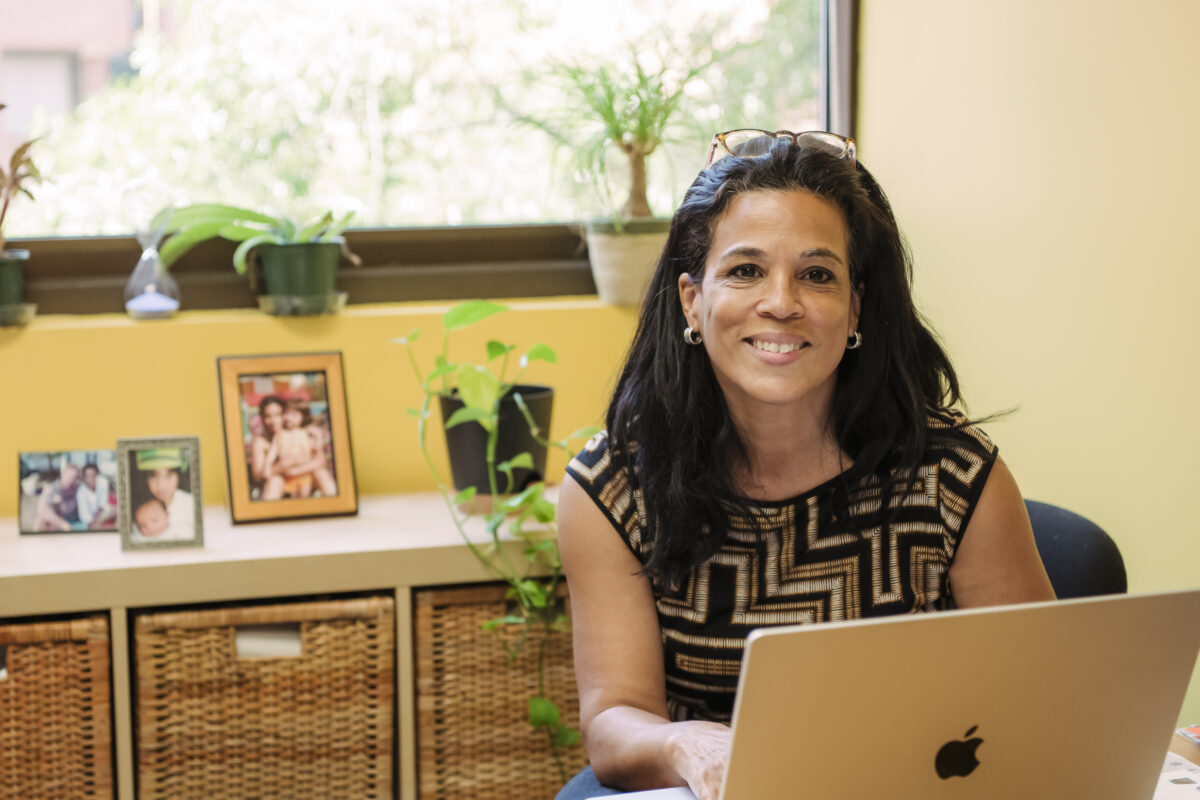
A long and winding road
To check whether NDRG1a actually did regulate this ATPase, her team tested how normal zebrafish and zebrafish without functional NDRG1a performed with normal oxygen and no oxygen. Under normal conditions, they both behaved normally. But the zebrafish without NDRG1a died at much higher rates without oxygen, providing compelling evidence that NDRG1a is required for the fish to adapt to low oxygen.
That was exciting, because at that time, “NDRG had no distinctive regions that could help researchers infer its function,” Brewster says—in fact, its molecular function was hardly understood. “But the fact that it can bind to a lot of different proteins suggests that it can function as an “adapter protein.” Just like an adapter that allows you to connect a USB to a wall outlet, adapter proteins connect molecules that otherwise wouldn’t interact. In this case, NDRG1a connects the ATPase with proteins that carry it off either to be stored in the cell for later use or destroyed—both outcomes that stop its activity and eliminate its demand for energy.
Brewster’s lab then showed that NDRG1a can, indeed, bind directly to the ATPase. Plus, the interaction increases when there is more lactate present, independent of how much oxygen is available. “That was really exciting for us,” Brewster says, “because it suggests that the rise in lactate is functioning via NDRG to promote the downregulation of ATPase.”
An important implication of this finding is that lactate or a similarly-shaped molecule could potentially be used to alter NDRG1a’s activity, and therefore artificially induce an energy-conserving state, Brewster explains. That could potentially help retain organs in viable condition for longer between collection and transplant—and that could save lives.
To further demonstrate that point, Brewster’s lab showed that if cells don’t have functional NDRG1a, ATPase activity does not decrease even with high levels of lactate. That means lactate doesn’t regulate ATPase on its own, but requires NDRG1a as an intermediary.
Joy in the process
Based on all the evidence they’ve collected—in their own lab via experiments and through research in the literature and genetic databases—the Brewster lab’s current model of the system is this: When oxygen is low, lactate and NDRG1a increase. Lactate binds to NDRG1a in such a way that allows it to interact with the ATPase, guiding it either to storage or to the cell’s “garbage can,” which improves the organism’s ability to survive low oxygen by reducing demand from the ATPase for energy.
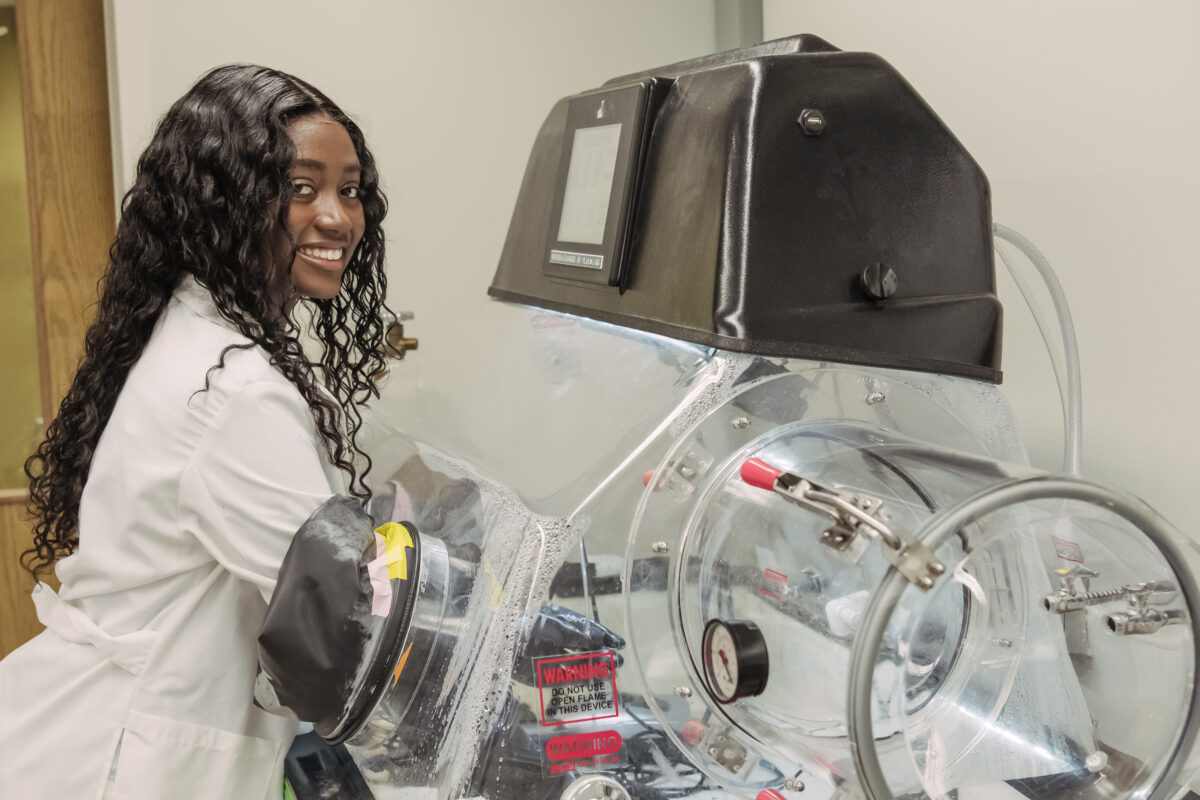
While it might sound complicated (and sometimes it is!), this process is the joy of a biologist—untangling how molecules interact, using logic to define long chains of reactions, and designing laboratory experiments to fill in knowledge gaps. It’s incremental work like Brewster’s that takes science to new heights step by step. Brewster’s research group has already meaningfully moved the needle on understanding how organisms adapt to oxygen deprivation, but there is still so much more to do—and Brewster is wasting no time.
Filling in the picture
“While hypoxia is damaging, what is even more damaging is the return to normal oxygen,” she says. “You cannot be hypoxia tolerant if you are not also tolerant to reoxygenation. That had us wondering if NDRG is also involved in the reoxygenation phase.”
Brewster’s group has determined that as oxygen returns, NDRG1a continues to interact with the ATPase. That suggests NDRG1a is also important for the transition to reoxygenated conditions. The next experiment the team needs to do to determine whether NDRG1a is indeed critical for reoxygenation is complicated: They need to allow zebrafish to experience hypoxia with normal NDRG1a, and then remove functional NDRG1a just prior to reoxygenation.
“And that is not a trivial experiment,” Brewster says. “So that is one big aim of the new NIH proposal—to try to address the undoing of the hypoxia response.”
Another big goal of Brewster’s upcoming work is to look at other proteins that NDRG1a interacts with and explore their potential roles in hypoxia response. She also wants to investigate other members of the NDRG family. For example, muscle cells produce more NDRG1b under hypoxia, which occurs during intense exercise along with an increase in lactate. Ph.D. candidate Prableen Chowdhary is investigating NDRG1b’s role now. And other NDRG family members are known to be abundant in tissues that require a lot of energy, like the heart.
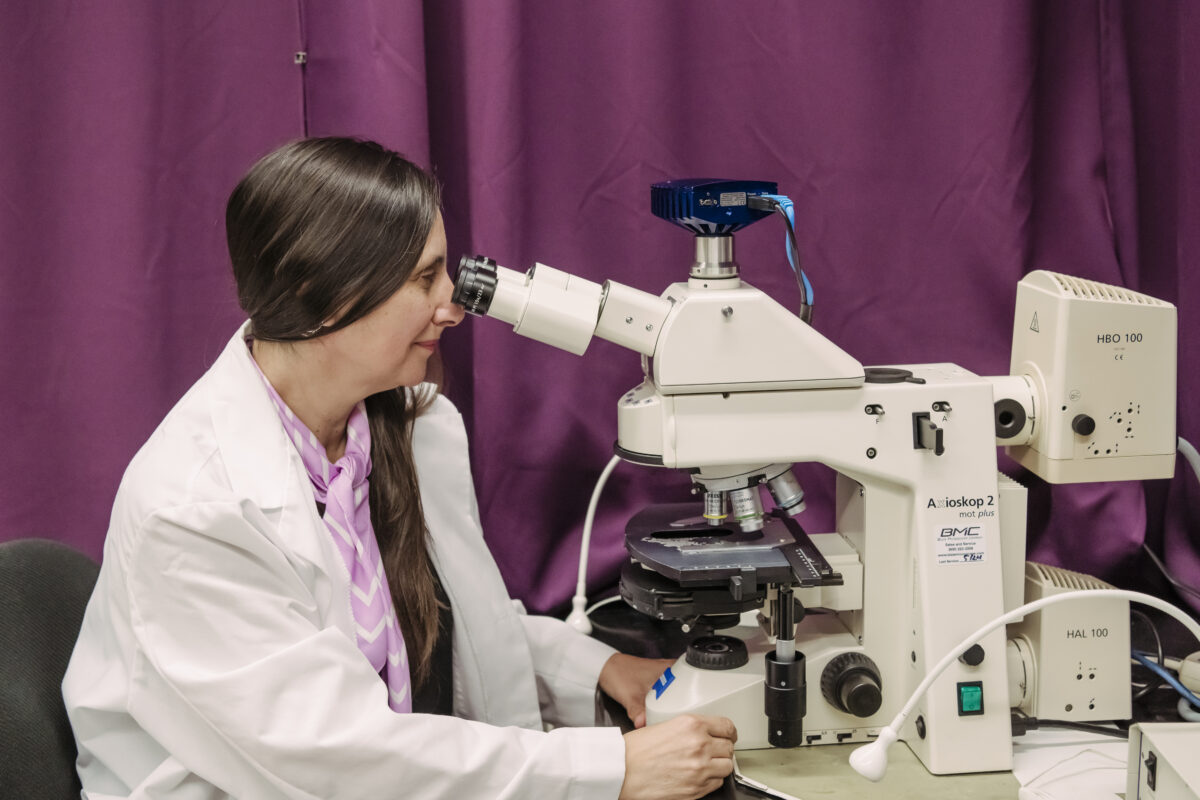
“It takes energy to save energy”
Curiously, Brewster also noticed that in the research literature, hypoxia is associated with hearing loss: Pilots, people who live at high altitudes, and people with oxygen-depleting diseases like sickle cell anemia or chronic sleep apnea all suffer from hearing loss at higher-than-average rates. Even more intriguingly, NDRG1 is expressed in the inner ear during hypoxia, and Ph.D. candidate Lilian Gonzalez is currently studying its role.
“NDRG seems to be protective, so it may be protective in the inner ear just like it is in the kidney and ionocytes,” Brewster says. “It might be down-regulating ATPase somehow to preserve those cells.”
Beyond NDRG, one of Brewster’s former students, Tim Hufford, Ph.D. ’23, biological sciences, discovered that cells begin producing larger quantities of many different proteins when oxygen drops. That finding officially sunk Brewster’s original hypothesis that metabolites, rather than new proteins, must be the first responders when reduced oxygen strikes. It also demonstrated that “it takes energy to save energy,” she says.
Hufford’s work “opened up a huge area of research, as we now know of hundreds of additional molecules that may play a critical role in hypoxia adaptation in addition to NDRGs,” Brewster says. “It’s more research than my lab could pursue in a lifetime,” she adds, encouraging the next generation to pick up the torch.
Finding purpose in student mentoring
Much fascinating research is in Brewster’s future—but she is far from alone in the endeavor. Brewster’s students are the drivers of the research, she says, and with the new grant, she might add a postdoctoral fellow as well.
“I am deeply committed to the students’ success,” Brewster says. More and more undergraduates in her laboratory are authoring academic papers, which will give them a leg up as they apply to graduate school or seek careers.
As a full professor, Brewster plans to dedicate her time at UMBC to “doing more of what I passionately care about,” she says. “And providing opportunities for people to truly excel in science is completely linked to the research. Because they are the people doing the research. And the students in my lab have done great things.”
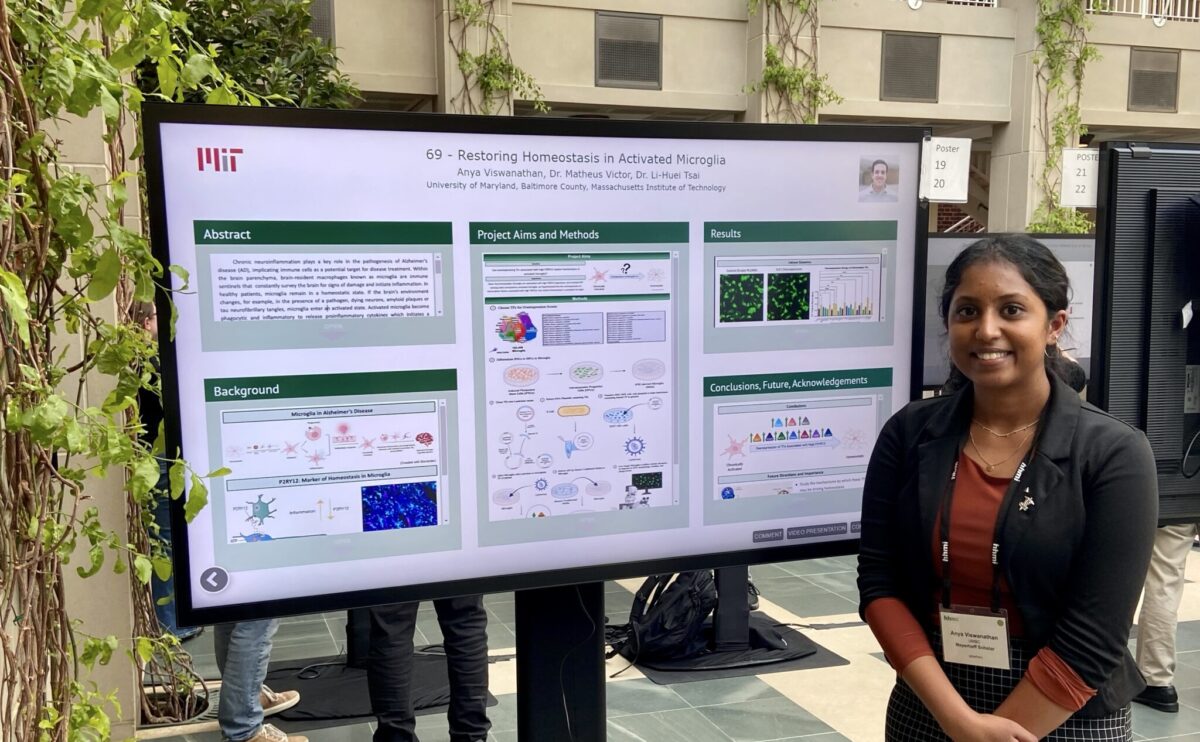
She’s not exaggerating. Gabriel Otubu, a senior biochemistry major in Brewster’s lab, received the prestigious Goldwater Scholarship earlier this year. Last year, Soujanya “Anya” Viswanathan ’24, biological sciences, was also named a Goldwater scholar as was Dominique Brooks ’21, biological sciences, in 2019. Brewster also mentors graduate students as director of the G-RISE Program, an NIH-funded initiative that offers mentorship and training for STEM careers in academia, industry, or government to graduate students from a wide range of backgrounds.
It’s this commitment to mentoring that underpins Brewster’s research success, and she also sees it as one of her fundamental roles as a faculty member. “I want to be very intentional with the time I have left, and student training is something I care deeply about,” she says. “Anyone can be excellent in science. And that is what I strive for in my lab—to support every student to reach their potential, no matter where they’re coming from.”