Deepa Madan, an assistant professor of mechanical engineering at UMBC, has received a prestigious National Science Foundation (NSF) CAREER award for research on materials that could improve wearable medical devices, reduce energy waste, and power sensors to monitor everything from the safety of infrastructure to the paths pollutants travel.
The roughly $500,000 grant, given over five years, will further Madan’s research on materials called thermoelectrics, which can turn a temperature difference into electricity, or vice versa.
“It’s a fantastic feeling to be recognized with this award, but I know that it is just the beginning,” says Madan. “The coming years will be an opportunity not only to meet the research goals, but also to contribute to public outreach and to give diverse students opportunities to connect to the research.”
“CAREER awards are one of the most significant awards at NSF for researchers,” says Erin Lavik, professor of chemical, biochemical, and environmental engineering. As associate dean for research and faculty development in the College of Engineering and Information Technology (COEIT), she helped COEIT researchers hone their NSF CAREER award applications to access more support and visibility for their high-impact work.
“Dr. Madan is absolutely brilliant, and I was so excited to hear that she got this award,” Lavik says. “It will allow her to build out her research and educational program in a way that aligns beautifully with where the next generation of materials needs to move to address environmental and sustainability concerns.”
Heat into electricity
Heat is all around us. It radiates from sweaty skin, pours out of hot car engines, and seeps out of sunbaked sidewalks at night. Thermoelectric materials tap into this ubiquitous form of energy, turning temperature differences into electrical current. (The materials can also run in reverse, using electricity to heat or cool.)
Thermoelectric materials can already be found in niche applications—such as chilling wine and powering spacecraft—but their more widespread adoption has been held back by their limited efficiency and rigid nature.
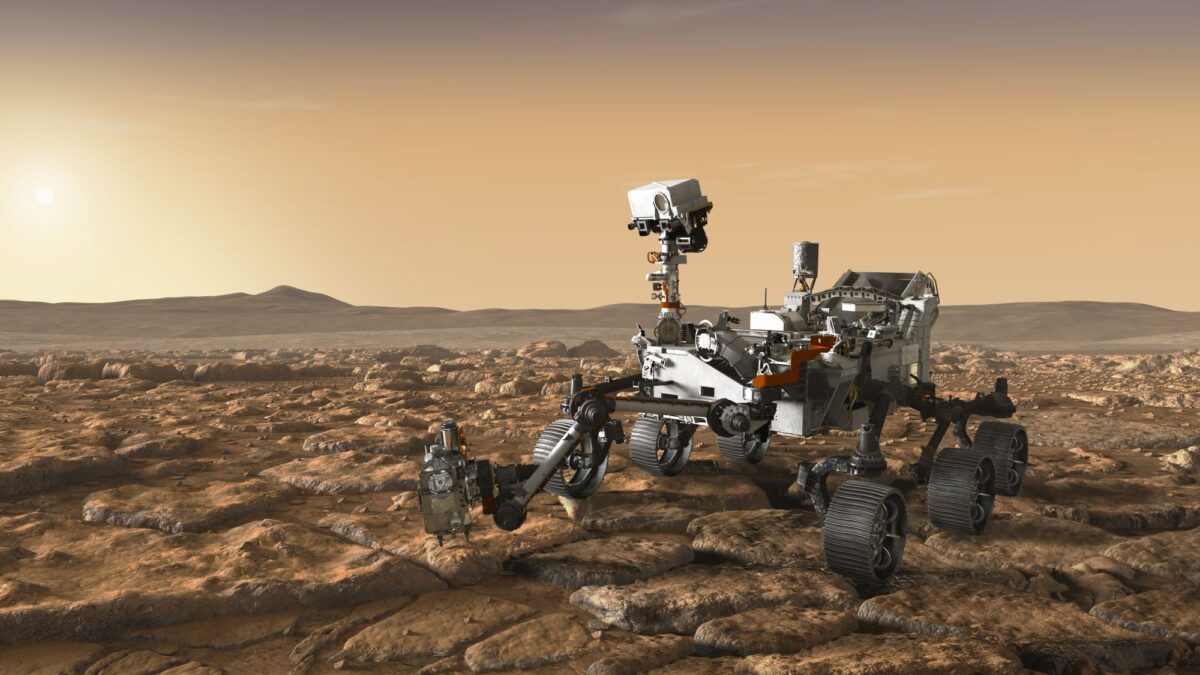
Madan’s research tackles both these challenges. She mixes grains of thermoelectric material with a pliable glue-like substance called a binder. The resulting composite material can be twisted and bent, making it more versatile. It’s also more affordable.
Adding the binder normally makes a thermoelectric material less efficient. In addition, when making a composite, researchers have historically baked the material at extremely high temperatures to harden the binder, which takes a lot of energy.
Madan is striving to keep her process efficient, low-energy, and environmentally friendly. At the same time, she is answering fundamental questions about the relationship between structure, process, and properties of her materials. That knowledge will help illuminate the best path toward further improving the materials’ properties.
Furthering fundamental science
Generally, when materials conduct electricity well, they also conduct heat well (think of the feel of a metal bench in winter). However, thermoelectrics require the opposite—they work best when they conduct electricity well, but conduct heat poorly. That way they can maintain the temperature difference that drives the electrons.
Madan’s research group aims to make materials optimized with high electrical conductivity and low thermal conductivity by studying the underlying science.
To start, they mix a small amount of a binder made from the shells of crustaceans with grains of a thermoelectric material called bismuth antimony telluride. Some of the thermoelectric grains are about the size of pollen grains, while others are thousands of times smaller. They form the mixture into the desired shape using a 3D printer, and then squeeze it together while applying gentle heat.
The researchers then study how each step affects the microscopic structure of the material and, consequently, its properties.
So far, they have found that the relatively large grains squeezed together provide a path for electrons to travel through the material, boosting its electrical conductivity. Meanwhile the super tiny grains and small defects where the grains meet the binder disrupt the waves of molecular vibrations that transport heat, keeping the thermal conductivity low.
Powering outreach
Ultimately, Madan hopes her thermoelectrics will power environmentally friendly devices of the future, but also drive students to become interested in the world of materials science. As part of her project, she will create a new class on flexible electronics and recruit high school and community college student researchers to work in her lab, in addition to her current UMBC students. She will also create an outreach kit for middle and high school students to make their own thermoelectrically-powered devices.
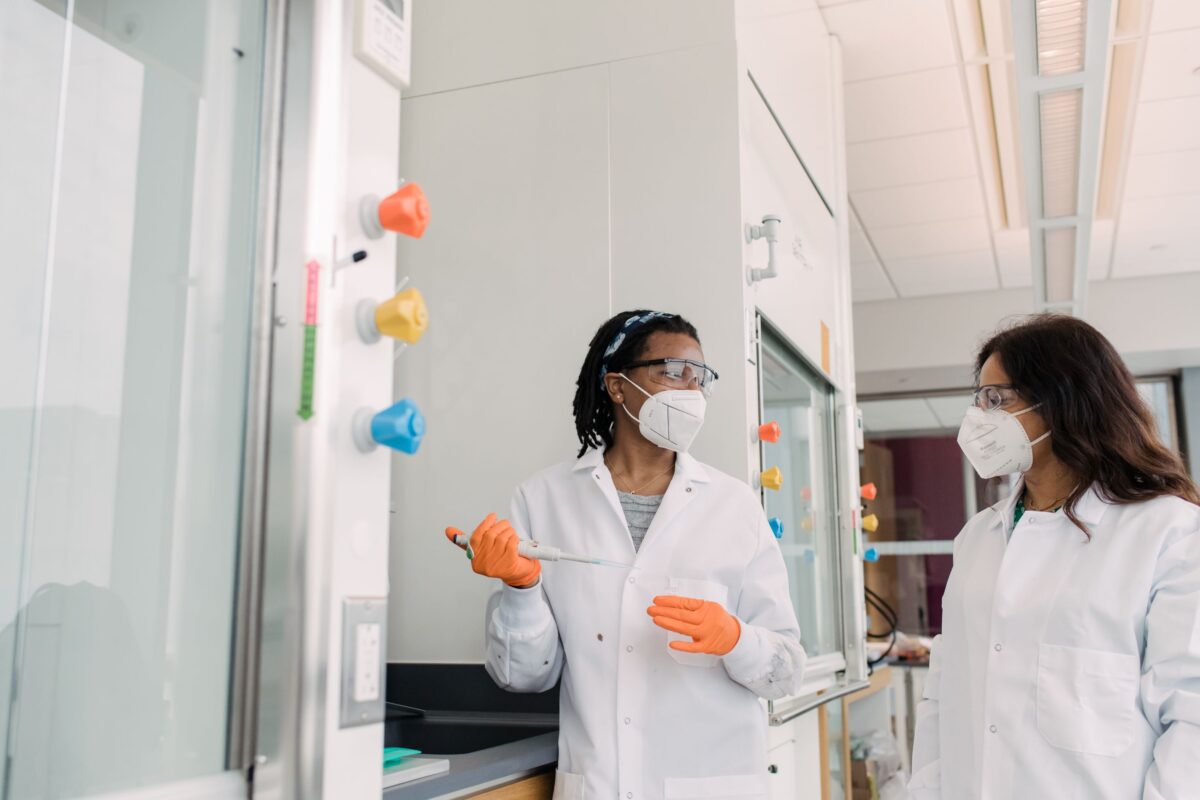
“I’m very passionate about education and outreach and UMBC gives us immense opportunities to connect with diverse populations,” says Madan. “I’m hoping with this award I can involve more students, especially women, who will get passionate about changing the world through STEM.”
Tags: COEIT, MechE, NSF CAREER award, rca-1, Research